ENERGY SUPPLY OF THE HEART AND SKELETAL MUSCLES DURING EXERCISE: MITOCHONDRIAL VECTOR
L.M. Gunina1, I.F. Belenichev2, K.V. Rosova3, Yu.O. Ataman4, V.L. Voitenko4, V.V. Bezugla1
- National Ukraine University of Physical Education and
Sport, Kyiv, Ukraine
- Zaporizhia Medical University, Zaporizhia, Ukraine
- Bogomolets Institute of Physiology of National Academy of Sciences of Ukraine, Kyiv, Ukraine
- Sumy State University, Sumy, Ukraine
DOI: https://doi.org/10.15407/fz68.05.067
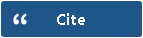
Abstract
In this review, we consider metabolic pathways of energy production from the standpoint of determining the mechanisms of
energy supply during intense physical activity and the need to
accelerate the process of ATP resynthesis; possible directions
of this process intensification are outlined. The most important
systems for an athlete to limit his physical performance are the
cardiovascular and muscular. That is why we emphasize on
energy production in myocardial cells and myocytes. Energy
production and energy exchange in cells is carried out by
mitochondria, which are the main organelles of energy supply. Functional activity of these organelles is provided by the
inner membrane, which contains components of the electron
transport chain and ATPase. Physical activity requires ATP
resynthesis, which is provided by different types of energyreleased reactions. An important part of our review reveals the
analysis of data on the mitochondria themselves, which are key
determinants of the functional state of the body’s cells during
physical activity. Functional consequences of the changes in
mitochondrial structure are of quite importance, especially
associated with different configurations of mitochondria and
mitochondrium − increasing the number of organelles and
crystal density, normalization of the structural and functional
state of the inner membrane and its protection from oxidative
stress which is inherent in the intense physical activity. Oxidative stress and working hypoxia are a very common cause
of further metabolic disorders, even before the formation of
hypertrophy of chronic physical exertion, when you have
to remove an athlete from the training process. In general,
numerous metabolic processes in the myocardium, which are
inherent in intense physical activity, undergo negative changes
with further activation of athletes. One of the ways to correct
energy deficiency caused by prolonged and intense physical
activity should be the use of exogenous or endogenous substances involved in energy metabolism.
Keywords:
physical activity; heart; energy; mitochondria; myocardial cells; skeletal muscles; ATP; hypoxia.
References
- Luft R. The development of mitochondrial Medicine. Proc Natl Acad USA. 1994;(91):8731-8.
CrossRef
PubMed PubMedCentral
- van der Bliek AM, Sedensky MM, Morgan PG. Cell biology of the mitochondrion. Genetics. 2017;207(3):843-71.
CrossRef
PubMed PubMedCentral
- Melnychuk SD, Khyzhnyak SV, Morozova VS, Stepanova LI, Uman JSC, Wojciech VM. Energy function of mitochondria of rat cardiomyocytes in artificial hypobiosis. Fiziol Zh. 2015;61(2):18-22.
- Belyaev NG. Structural changes in muscle fiber during the period of adaptation to physical activity of varying intensity. Nauka. Ynnovatsyy Tekhnol. 2014;1(5):180-8. [Russian].
- Rozova KV, Bolgova TV, Tymoshenko KR, Vinnychuk YuD, Gunina LM, Bezugla VV. Reconstruction of skeletal muscle tissues, lungs of rats under conditions of stress hypoxia in the experiment. Fiziol Zh. 2016;62(6):72-80. [Ukrainian].
CrossRef
PubMed
- Henze, K, Martin W. Evolutionary biology: Essence of mitochondria. Nature. 2003;426(6963):127-8.
CrossRef
PubMed
- Voitenko VL, Gunina LM. Infusion of burstinic acid on the change of the mitochondrial apparatus of skeletal malignancies in the modeling of physical changes in the experiment. Ukr Zh Med Biol Sportu. 2021;6(1/29):293-302. [Ukrainian].
CrossRef
- Koch RE, Josefson CC, Hill GE. Mitochondrial function, ornamentation, and immunocompetence. Biol Rev Camb Philos Soc. 2017;92(3):1459-74.
CrossRef
PubMed
- Kühlbrandt W. Structure and function of mitochondrial membrane protein complexes. BMC Biol. 2015;13:89.
CrossRef
PubMed PubMedCentral
- Abate M, Festa A, Falco M, Lombardi A, Luce A, Grimaldi A, Zappavigna S, Sperlongano P, Irace C, Caraglia M, Misso G. Mitochondria as playmakers of apoptosis, autophagy and senescence. Semin Cell Dev Biol. 2020;98:139-53.
CrossRef
PubMed
- Kondrashova MN. Succinic acid is a source of energy in the body. Norma-press. 1991;9:17-8. [Russian].
- Vdovenko N, Ivanova A, Khrobatenko O. The use of nanotechnology in the development of special foods for athletes. Aktual Probl Fizych Kultury Sportu. 2016;35(1):11-7. [Ukrainian].
- Corciulo C, Lendhey M, Wilder T, Schoen H, Cornelissen AS, Chang G, Kennedy OD, Cronstein BN. Endogenous adenosine maintains cartilage homeostasis and exogenous adenosine inhibits osteoarthritis progression. Nat Commun. 2017;8:15019.
CrossRef
PubMed PubMedCentral
- Sarkar S, Mondal J. Mechanistic insights on ATP's role as hydrotrope. J Phys Chem B. 2021;125(28):7717-31.
CrossRef
PubMed
- Vigh-Larsen JF, Ørtenblad N, Spriet LL, Overgaard K, Mohr M. Muscle glycogen metabolism and high-intensity exercise performance: A narrative review. Sports Med. 2021;51(9):1855-74.
CrossRef
PubMed
- Hargreaves M, Spriet LL. Skeletal muscle energy metabolism during exercise. Nat Metab. 2020;2(9):817-28.
CrossRef
PubMed
- Seaborne RA, Sharples AP. The interplay between exercise metabolism, epigenetics, and skeletal muscle remodeling. Exerc Sport Sci Rev. 2020;48(4):188-200.
CrossRef
PubMed
- Naini A, Gilkerson R, Shanske S, Pang J. Detection of mitochondrial DNA (mtDNA) mutations. Methods Cell Biol. 2020;155:383-400.
CrossRef
PubMed PubMedCentral
- Wu Z, Oeck S, West AP, Mangalhara KC, Sainz AG, Newman LE, Zhang XO, Wu L, Yan Q, Bosenberg M, Liu Y, Sulkowski PL, Tripple V, Kaech SM, Glazer PM, Shadel GS. Mitochondrial DNA Stress signalling protects the nuclear genome. Nat Metab. 2019;1(12):1209-18.
CrossRef
PubMed PubMedCentral
- Zhang Y, Wong HS. Are mitochondria the main contributor of reactive oxygen species in cells? J Exp Biol. 2021;224(Part 5):jeb221606.
CrossRef
PubMed
- Holloszy JO. Regulation by exercise of skeletal muscle content of mitochondria and GLUT4. J Physiol Pharmacol. 2008;59(Suppl 7):5-18.
- Granata C, Jamnick NA, Bishop DJ. Training-induced changes in mitochondrial content and respiratory function in human skeletal muscle. Sports Med. 2018;48(8):1809-28.
CrossRef
PubMed
- Valero T. Mitochondrial biogenesis: pharmacological approaches. Curr Pharm Des. 2014;20(35):5507-9.
CrossRef
PubMed
- Glancy B, Kim Y, Katti P, Willingham TB. The functional impact of mitochondrial structure across subcellular scales. Front Physiol. 2020;11:541040.
CrossRef
PubMed PubMedCentral
- Marín-García J, Akhmedov AT. Mitochondrial dynamics and cell death in heart failure. Heart Fail Rev. 2016; 21(2):123-36.
CrossRef
PubMed
- Ma M, Chen W, Hua Y, Jia H, Song Y, Wang Y. Aerobic exercise ameliorates cardiac hypertrophy by regulating mitochondrial quality control and endoplasmic reticulum stress through M2 AChR. J Cell Physiol. 2021;236(9):6581-96.
CrossRef
PubMed
- Miranda-Silva D, Rodrigues GP, Alves E, Rizo D, Fonseca ACRG, et al. Mitochondrial reversible changes determine diastolic function adaptations during myocardial (reverse) remodeling. Circ Heart Fail. 2020;13(11):e006170.
CrossRef
PubMed
- Rongjun Zou, Jun Tao, Junxiong Qiu, Wanting Shi, Minghui Zou, Weidan Chen, et al. Ndufs1 deficiency aggravates the mitochondrial membrane potential dysfunction in pressure overload-induced myocardial hypertrophy. Oxid Med Cell Longev. 2021;2021:5545261.
CrossRef
PubMed PubMedCentral
- Moaddel R, Ubaida-Mohien C, Tanaka T, Lyashkov A, Basisty N, Schilling B, et al. Proteomics in aging research: A roadmap to clinical, translational research. Aging Cell. 2021;20(4):e13325.
CrossRef
PubMed PubMedCentral
- Salazar-Ramírez F, Ramos-Mondragón R, GarcíaRivas G. Mitochondrial and sarcoplasmic reticulum interconnection in cardiac arrhythmia. Front Cell Dev Biol. 2021;8:623381.
CrossRef
PubMed PubMedCentral
- Cadenas S. Mitochondrial uncoupling, ROS generation and cardioprotection. Biochim Biophys Acta Bioenerg. 2018;1859(9):940-50.
CrossRef
PubMed
- Adlam VJ, Harrison JC, Porteous CM, et al. Targeting an antioxidant to mitochondria decreases cardiac ischemiareperfusion injury. FASEB J. 2005;19(9):1088-95.
CrossRef
PubMed
- Song R, Dasgupta C, Mulder C, Zhang L. MicroRNA-210 Controls mitochondrial metabolism and protects heart function in myocardial infarction. Circulation. 2022;145(15):1140-53.
CrossRef
PubMed
- Jiang Q, Yin J, Chen J, Ma X, Wu M, Liu G, et al. Mitochondria-targeted antioxidants: A step towards disease treatment. Oxid Med Cell Longev. 2020;2020:8837893.
CrossRef
PubMed PubMedCentral
- Horikoshi Y, Yan Y, Terashvili M, Wells C, Horikoshi H, et al. Fatty acid-treated induced pluripotent stem cell-derived human cardiomyocytes exhibit adult cardiomyocyte-like energy metabolism phenotypes. Cells. 2019;8(9):1095.
CrossRef
PubMed PubMedCentral
- Frayn KN The glucose-fatty acid cycle: a physiological perspective. Biochem Soc Trans. 2003;31(6):1115-9.
CrossRef
PubMed
- Bianchi VE. Impact of nutrition on cardiovascular function. Curr Probl Cardiol. 2020;45(1):100391.
CrossRef
PubMed
- Fritzen AM, Lundsgaard AM, Kiens B. Tuning fatty acid oxidation in skeletal muscle with dietary fat and exercise. Nat Rev Endocrinol. 2020;16(12):683-96.
CrossRef
PubMed
- Cruz MM, Lopes AB, Crisma AR, de Sá RCC, Kuwabara WMT, Curi R, et al. Palmitoleic acid (16:1n7) increases oxygen consumption, fatty acid oxidation and ATP content in white adipocytes. Lipids Health Dis. 2018;17(1):55.
CrossRef
PubMed PubMedCentral
- Tsapko LP, Afanasiev SA, Maksimov IV. Prospects for metabolic therapy in heart disease. Siberian Med J. 2016;31(4):7-12.
- Glancy B. Visualizing mitochondrial form and function within the cell. Trends Mol Med. 2020;26(1):58-70.
CrossRef
PubMed PubMedCentral
- Rozova EV, Mankovskaya IN, Mironova GD. Structural and dynamic changes in rat myocardial mitochondria during acute hypoxic hypoxia: the role of the mitochondrial ATP-dependent potassium channel. Biochimija. 2015;80(8):1186-1194. [Ukrainian].
CrossRef
PubMed
- Cardoso AC, Lam NT, Savla JJ, Nakada Y, Pereira AHM, Elnwasany A, et al. Mitochondrial substrate utilization regulates cardiomyocyte cell cycle progression. Nat Metab. 2020;2(2):167-78.
CrossRef
PubMed PubMedCentral
- Makarewich CA, Baskin KK, Munir AZ, Bezprozvannaya S, Sharma G, Khemtong C, et al. MOXI Is a mitochondrial micropeptide that enhances fatty acid β-oxidation. Cell Rep. 2018;23(13):3701-9.
CrossRef
PubMed PubMedCentral
- Wang Y, Wei L, Wei D, Li X, Xu L, Wei L. Testisspecific lactate dehydrogenase (LDH-C4) in skeletal muscle enhances a pika's sprint-running capacity in hypoxic environment. Int J Environ Res Publ Health. 2015;12(8):9218-36.
CrossRef
PubMed PubMedCentral
- Olesova VM, Markatyuk OYu, Yurova YuYu. Myocardial metabolism and drugs of metabolic action. Cardiologija. 2013;53(1):66-71. [Russian].
- Kapilevich LV, Dyakova EY, Kabachkova AV. Sports biochemistry with the basics of sports pharmacology: Textbook. Publ House Tomsk Univ, 2010. [Russian].
- Adeva-Andany MM, Calvo-Castro I, FernándezFernández C, Donapetry-García C, Pedre-Piñeiro AM. Significance of L-carnitine for human health. IUBMB Life. 2017;69(8):578-94.
CrossRef
PubMed
- Golovach IU, Avramenko OM. The use of metabolic correctors based on meldonium dihydrate: a modern approach in the combination therapy of coronary heart disease. Med Ukr. 2011;8(154):68-72. [Ukrainian].
- Mazur I, Belenichev I, Kucherenko L, Bukhtiyarova N, Puzyrenko A, Khromylova O, et al. Antihypertensive and cardioprotective effects of new compound 1-(β-phenylethyl)-4-amino-1,2,4-triazolium bromide (Hypertril). Eur J Pharmacol. 2019;853:336-44. [Ukrainian].
CrossRef
PubMed
- Belenichev I, Gorbachova S, Pavlov S, Bukhtiyarova N, Puzyrenko A, Brek O. neurochemical status of nitric oxide in the settings of the norm, ishemic event of central nervous system, and pharmacological Bn intervention. Georg Med News. 2021;(315):169-76.
- Ramana KV, Srivastava S, Singhal SS. Lipid peroxidation products in human health and disease 2016. Oxid Med Cell Long. 2017:2163285.
CrossRef
PubMed PubMedCentral
- Raymer GH, Green HJ, Ranney DA. Muscle metabolism and acid-base status during exercise in forearm workrelated myalgia measured with 31P-MRS. J Appl Physiol. 2009;106(4):1198-206.
CrossRef
PubMed
- Sciarretta S, Maejima Y, Zablocki D, Sadoshima J. The role of autophagy in the heart. Annu Rev Physiol. 2018;80:1-26.
CrossRef
PubMed
- Shi B, Ma M, Zheng Y, Pan Y, Lin X. mTOR and Beclin1: Two key autophagy-related molecules and their roles in myocardial ischemia/reperfusion injury. J Cell Physiol. 2019;234(8):12562-68.
CrossRef
PubMed
- Bezugla VV, Rozova KV, Vinnychuk YuD. Differences in structural changes of the myocardium during acute and prolonged exercise in the experiment. Ukr Zh Med Biol Sportu. 2017;(1):120-5. [Ukrainian].
CrossRef
- Lakhal-Littleton S, Robbins PA. The interplay between iron and oxygen homeostasis with a particular focus on the heart. J Appl Physiol. 2017;123(4):967-73.
CrossRef
PubMed PubMedCentral
- Yuan X, Braun T. Multimodal regulation of cardiac myocyte proliferation. Circ Res. 2017;121(3):293-309.
CrossRef
PubMed
- Yoshida Y, Shimizu I, Minamino T. Capillaries as a therapeutic target for heart failure. J Atheroscler Thromb. 2022 Apr 1.
CrossRef
PubMed PubMedCentral
- Thonusin C, Pantiya P, Sumneang N, Chunchai T, Nawara W, Arunsak B, Siri-Angkul N, Sriwichaiin S, Chattipakorn SC, Chattipakorn N. Effectiveness of high cardiorespiratory fitness in cardiometabolic protection in prediabetic rats. Mol Med. 2022;28(1):31.
CrossRef
PubMed PubMedCentral
- Mikhalyuk EL, Sivolap VV. Features of the electrocardiogram of persons engaged in sports. Message I (a review). Zaporiz Med Zh. 2019;21/2(113):264-9. [Ukrainian].
- Bernardi L. Interval hypoxic training. Adv Exp Med Biol. 2001;502:377-99.
CrossRef.1007/978-1-4757-3401-0_25
PubMed
- Huang YC, Hsu CC, Wang JS. High-intensity interval training improves erythrocyte osmotic deformability. Med Sci Sports Exerc. 2019;51(7):1404-12.
CrossRef
PubMed
- Gavenauskas BL, Mankovskaya IM, Nosar VI, Nazarenko AI. The effect of interval hypoxic training on the rates of adaptation of rats to stress hypoxia. Fiziol Zh. 2004;50(6):32-42. [Ukrainian].
- Abe H, Semba H, Takeda N. the roles of hypoxia signaling in the pathogenesis of cardiovascular diseases. J Atheroscler Thromb. 2017;24(9):884-94.
CrossRef
PubMed PubMedCentral
- Poluektov YM, Petrushanko IY, Undrovinas NA, Lakunina VA, Khapchaev AY, Kapelko VI, et al. Glutathionerelated substances maintain cardiomyocyte contractile function in hypoxic conditions. Sci Rep. 2019;9(1):4872.
CrossRef
PubMed PubMedCentral
- Gatrell LA, Farhat E, Pyle WG, Gillis TE. Contractile function of the excised hagfish heart during anoxia exposure. J Comp Physiol B. 2019;189(2):199-211.
CrossRef
PubMed
- Hargreaves M, Spriet LL. Exercise metabolism: Fuels for the fire. Cold Spring Harb Perspect Med. 2018; 8(8):a029744.
CrossRef
PubMed PubMedCentral
- Gunina LM. Influence of succinic acid and its derivatives on the physical performance of athletes. Dop NANU. 2013;(3):180-4. [Ukrainian].
- Philpott JD, Witard OC, Galloway SDR. Applications of omega-3 polyunsaturated fatty acid supplementation for sport performance. Res Sports Med. 2019;27(2):219-37.
CrossRef
PubMed
- Slater GJ, Sygo J, Jorgensen M. SPRINTING. Dietary approaches to optimize training adaptation and performance. Int J Sport Nutr Exerc Metab. 2019; 29(2):85-94.
CrossRef
PubMed
- Belenichev IF, Gorbacheva SV, Demchenko AV, Bukhtiyarova NV. The thiol-disulfide balance and the nitric oxide system in the brain tissue of rats subjected to experimental acute impairment of cerebral blood flow: The therapeutic effects of nootropic drugs. Neurochem J. 2014;8:24-7.
CrossRef
- Velenichev IF, Kolesnik YM, Pavlov SV, Sokolik EP, Bukhtiyarova NV. Malate-aspartate shunt in neuronal adaptation to ischemic conditions: Molecular-biochemical mechanisms of activation and regulation. Neurochem J. 2012;6:22-8.
CrossRef
- Ke Q, Costa M. Hypoxia-inducible factor-1 (HIF-1). Mol Pharmacol. 2006;70(5):1469-80.
CrossRef
PubMed
- Fong GH. Mechanisms of adaptive angiogenesis to tissue hypoxia. Angiogenesis. 2008;11(2):121-40.
CrossRef
PubMed
- Korbecki J, Simińska D, Gąssowska-Dobrowolska M, Listos J, Gutowska I, Chlubek D, Baranowska-Bosiacka I. Chronic and cycling hypoxia: Drivers of cancer chronic inflammation through HIF-1 and NF-κB activation: A review of the molecular mechanisms. Int J Mol Sci. 2021;22(19):10701.
CrossRef
PubMed PubMedCentral
- Yao G, Zhang Q, Doeppner TR, Niu F, Li Q, Yang Y, Kuckelkorn U, Hagemann N, Li W, Hermann DM, Dai Y, Zhou W, Jin F. LDL suppresses angiogenesis through disruption of the HIF pathway via NF-κB inhibition which is reversed by the proteasome inhibitor BSc2118. Oncotarget. 2015;6(30):30251-62.
CrossRef
PubMed PubMedCentral
- Weinstein PR, Hong S, Sharp FR. Stroke. Molecular identification of the ischemic penumbra. 2004;35(11 Suppl 1):2666-70.
CrossRef
PubMed
- Date T, Mochizuki S, Belanger AJ, Yamakawa M, Luo Z, Vincent KA, Cheng SH, Gregory RJ, Jiang C. Expression of constitutively stable hybrid hypoxia-inducible factor1alpha protects cultured rat cardiomyocytes against simulated ischemia-reperfusion injury. Am J Physiol Cell Physiol. 2005;288(2):C314-20.
CrossRef
PubMed
- Agrawal M, Kumar V, Singh AK, Kashyap MP, Khanna VK, Siddiqui MA, Pant AB. Trans-resveratrol protects ischemic PC12 cells by inhibiting the hypoxia associated transcription factors and increasing the levels of antioxidant defense enzymes. ACS Chem Neurosci. 2013;4(2):285-94.
CrossRef
PubMed PubMedCentral
- Belenichev IF, Kolesnik YM, Pavlov SV, Sokolik EP, Bukhtiyarova NV. Disturbance of HSP70 chaperone activity is a possible mechanism of mitochondrial dysfunction. Neurochem J. 2011;5:251-6.
CrossRef
- Belenichev IF, Mazur IA, Kucherenko LI, Nagornaya EA, Gorbacheva SV, Bidnenko AS. The molecular and ultrastructural aspects of the formation of mitochondrial dysfunction in the modeling of chronic cerebral ischemia: The mitoprotective effects of angiolin. Neurochem J. 2016;10:131-6.
CrossRef
- Belenichev IF, Egorov AA. Synergism of the pharmacological effect of glycine and thiotriazoline. Pathology. 2021;18(1/51):23-32.
CrossRef
|