Regulation of lipid peroxidation in mitochondria by nitroglycerine
O.V. Akopova, Yu.P. Korkach, V.F. Sagach
O.O. Bogomoletz Institute of Physiology of National Academy of Science of Ukraine, Kyiv, Ukraine
DOI: https://doi.org/10.15407/fz68.03.003
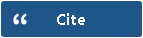
Abstract
The mechanisms of lipid oxidation under the application of nitroglycerine (NG) were studied in isolated
rat heart and liver mitochondria. Dose-dependent formation of diene conjugates (DC), leukotriene C4
(LTC4) and thromboxane B2 (TxB2) was shown. To disclose the mechanisms regulating lipid peroxidation in
mitochondria, we studied the effect of NG application on the formation of prooxidants (H2O2 and free Fe2+),
as well as xanthine oxidase and mtNOS activity as main sources of ROS and RNS. Based on the correlation
dependences, we have found that DC, LTC4, and TxB2 formation was strongly dependent on hydroperoxide
production and free divalent iron release in mitochondria. Also, DC formation exhibited the dependence
on Ca2+ uptake in mitochondria. No dependence of lipid oxidation on xanthine oxidase activity was found.
In heart, but not liver mitochondria, DC, LTC4, and TxB2 exhibited strong dependence on mtNOS activity,
but were independent of nitrosothiols formation. This indicated that lipid oxidation was independent of
direct protein nitrosylation caused by NG application. No dependence of lipid oxidation on mtNOS activity
in liver was found, which agreed with much higher mtNOS activity in heart mitochondria, and suppression
of mtNOS activity in liver mitochondria at high doses of NG. So, we came to the conclusion that under
NG application ROS overproduction and free Fe2+ release promoted both enzymatic and non-enzymatic
lipid oxidation in heart and liver mitochondria. Also, we hypothesized that RNS overproduction due to the
elevated mtNOS activity in the heart could largely contribute to lipid peroxidation and promote much faster
increase in the formation of lipid oxidation products in heart as compared to liver mitochondria, especially
at high doses of NG. Obtained correlation dependences allowed us disclose free iron, hydroperoxide, and
mtNOS activity as principal factors affecting lipid peroxidation in mitochondria under NG application.
Keywords:
nitroglycerine; heart; liver; mitochondria; ROS; RNS; lipid peroxidation; mtNOS
References
1. Andreadou I, Schulz R, Papapetropoulos R, Turan B, Ytrehus K, Ferdinandy P, Daiber A, Di Lisa F. The role of mitochondrial reactive oxygen species, NO and H2S in ischemia/reperfusion injury and cardioprotection. J Cell Mol Med. 2020; 24:6510-22.
2. Bibli S-I, Papapetropoulos A, Iliodromitis EK, et al. Nitroglycerine limits infarct size through S-nitrosation of cyclophilin D: a novel mechanism for an old drug. Cardiovascul Res. 2019;115:625-36.
3. Ignarro LJ, Napoli C, Loscalzo J. Nitric oxide donors and cardiovascular agents modulating the bioactivity of nitric oxide. Circ Res. 2002;90(1):21-8.
4. Daiber A, Wenzel P, Oelze M, et al. Mitochondrial aldehyde dehydrogenase (ALDH-2) – maker of and marker for nitrate tolerance in response to nitroglycerine treatment. Chem Biol Interact. 2009;178(1-3):40-7.
5. Akopova O, Kotsiuruba A, Korkach Y, Kolchinskaya L, Nosar V, Gavenauskas B, Serebrovska Z, Mankovska I, Sagach V. The effect of NO donor on calcium uptake and reactive nitrogen species production in mitochondria. Cell Physiol Biochem. 2016; 39(1):193-204.
6. Brookes PS. Mitochondrial nitric oxide synthase. Mitochondrion. 2004;3:187-204.
7. Reutov VP, Sorokina EG, Ohotin VE, Kositsyn NS. Cyclic transformations of nitric oxide in the organism of mammals. M.: Nauka. 1998. [Russian].
8. Ullrich V, Schildknecht S. Sensing hypoxia by mito- chondria: a unifying hypothesis involving s-nitrosation. Antioxid Redox Signal. 2014;20:325-38.
9. Akopova OV, Korkach YuP, Sagach VF. The regulation of mitochondrial NO synthase activity under nitroglycerine application in vivo. Fiziol Zh. 2022;68(1):3-12.
10. Radi R, Beckman JC, Bush KM, Freeman BA. Peroxy- nitrite-induced membrane lipid peroxidation: the cytotoxic potential of superoxide and nitric oxide. Arch Biochem Biophys. 1991;288:481-7.
11. Wang B, Wu L, Chen J, Dong L, Chen Ch, Wen Zh, Hu J, Fleming I, Wang DW. Metabolism pathways of arachidonic acids: mechanisms and potential therapeutic targets. Sig Transduct Target Ther. 2021; 6:94.
12. Leslie Ch C. Cytosolic phospholipase A2: physiological function and role in disease. J Lipid Res. 2015; 56:1386-402.
13. Moon SH, Jenkins ChM, Liu X, Guan Sh, Mancuso DJ, Gross RW. Activation of mitochondrial calcium-independent phospholipase A2γ (iPLA2γ) by divalent cations mediating arachidonate release and production of downstream eicosanoids. J Biol Chem. 2012;287:14880-95.
14. Jabůrek M, Ježek J, Zelenka J, Ježek P. Antioxidant activity by a synergy of redox-sensitive mitochondrial phospholipase A2 and uncoupling protein-2 in lung and spleen. Int J Biochem Cell Biol. 2013;45(4):816-25.
15. TyurinaYY, Poloyac SM, TyurinVA, Kapralov AA, Jiang J, Anthonymuthu TS, KapralovaVI, Vikulina AS, Jung Mi- Y, Epperly MW, Mohammadyani D, Klein-Seetharaman J, Jackson TC, Kochanek PM, Pitt BR, Greenberger JS, Vladimirov YA, Bayır H, Kagan VE. A mitochondrial pathway for biosynthesis of lipid mediators. Nat Chem. 2014 (6):542-52.
16. Gadd ME, Broekemeier KM, Crouser ED, Kumar J, Graff G, Pfeiffer DR. Mitochondrial iPLA2 activity modulates the release of cytochrome c from mitochondria and influences the permeability transition. J Biol Chem. 2006;281(11):6931-9.
17. Slatter DA, Aldrovandi M, O’Connor A, Allen SM, Brasher ChJ, Murphy RC, Mecklemann S, Ravi S, Daeley-Usmar V, O’Donnell VB. Mapping the human platelet lipidome reveals cytosolic phospholipase A2 as a regulator of mitochondrial bioenergetics during activation. Cell Metab. 2016. 23:1-15.
18. Gavrilov VB, Gavrilova AR, Hmara NF. Determination of diene conjugates in blood plasma by UV absorbance of heptane and isopropanol extracts. Lab Delo. 1988;60-4. [Russian].
19. Levine L, Alam I. Deacylation of cellular lipids and arachi- donic acid metabolism. Prog Lipid Res. 1981;20:81-8.
20. Huwiler M, Kohler H: Pseudo-catalytic degradation of hydrogen peroxide in the lactoperoxidase/H2O2/iodide system. Eur J Biochem 1984;141:69-74.
21. Buga GM, Singh R, Pervin S, Rogers NE, Schmitz DA, Jenkinson CP, Cederbaum SD, Ignarro LJ. Arginase activity in endothelial cells: inhibition by NG-hydroxy-L- arginine during high-output NO production. Am J Physiol 1996;271:H1988-98.
22. Korkach YuP, Dudchenko NO, Kotsiuruba AV. The role of non-heme iron in the protection effect of ecdysterone on the development of streptozotocin-induced hypoglycemia in rats. Ukr Biochem J. 2008;80(1):46-51.
23. Radi R, Turrens JF, Freeman BA. Cytochrome c-catalyzed membrane lipid peroxidation by hydrogen peroxide. Arch Biochem Biophys. 1991;288(1):118-25.
24. Bertero E, Maack Ch. Calcium signaling and reactive oxy- gen species in mitochondria. Circ Res. 2018;122:1460-78.
25. Hernansanz-Agustin P, Enriquez JA. Generation of reactive oxygen species by mitochondria. Antioxidants. 2021;10:415.
26. Berry CE, Hare JM. Xanthine oxidoreductase and car- diovascular disease: molecular mechanisms and patho- physiological implications. J Physiol. 2004;555:589-606.
27. Nazarewicz RR, Zenebe WJ, Parihar A, Parihar MS, Vaccaro M, Rink C, Sen CK, Ghafourifar P. 12(S)-hyd- roperoxyeicosatetraenoic acid (12-HETE) increases mitochondrial nitric oxide by increasing intramitochondrial calcium. Arch Biochem Biophys. 2007;468(1):114-20.
28. Pfeiffer S, Gorren ACF, Schmidt K, et al. Metabolic fate of peroxynitrite in aqueous solution. Reaction with nitric oxide and pH-dependent decomposition to nitrite and oxygen in 2:1 stoichiometry. J Biol Chem. 1997;272(6):3465-70.
|