Effect of various origins conditioned media on the migration of neural cells in vitro
L.D. Liubich1, L.P. Staino1, D.M. Egorova1, T.D. Skaterna2, E.G. Pedachenko1
- The State Institution “Romodanov Neurosurgery Institute, National Academy of Medical Sciences
of Ukraine”, Kyiv, Ukraine
- Palladin Institute of Biochemistry of the National Academy of Sciences of Ukraine, Kyiv, Ukraine
DOI: https://doi.org/10.15407/fz68.02.036
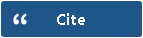
Abstract
An important direction in the development of the latest technologies for the restoration of damaged
central nervous system is the use of stem/progenitor cells (SPCs), mainly neurogenic SPCs (NSPCs) and
mesenchymal multipotent stromal cells (MMSCs). One of the main mechanisms of SPCs action is indirect
paracrine effects due to the ability to produce a wide range of biologically active signaling molecules
(secretome). The study of regenerative effects of conditioned media (CM) of NSPCs and MMSCs as a source
of their secretome seems to be actual and potentially beneficial. The aim of the study is to compare the
impact of CM from 24-h cultures of fetal neurogenic cells (NCs (E14), as a source of NSPCs) and adiposederived mononuclear cells (AMCs as a source of MMSCs) on migration capacity of rat neural cells in vitro.
AMCs-CM were obtained from 24-h cultures with prevalence of CD105+ cells and ability upon further
cultivation to form “spheroids” and potency to differentiate into different cell types. NCs-CM were
obtained from 24-h cultures with prevalence of Nestin+ cells and ability upon further cultivation to form
“neurospheres” and potency to differentiate into astrocytes (GFAP+) and neurons (β-Tubulin III+). Rat
fetal neural cells (E14) were cultured to achieve a confluent monolayer with basic cellular elements of
nervous tissue (5-7th day), which was dissected with forming a transection site and DMEM with 10% fetal
calf serum (control) or 0.1-0.3 mg/ml (by total protein amount) of NCs-CM or AMCs-CM were added. In
control cultures of rat neural cells partial overgrowth of the dissected area of the monolayer was observed
due to the migration of cells, formation of a network of processes and intercellular contacts; reaching 13.2%
(4th day) – 23.2% (8th day) of its full length. The overgrown area increased after addition of CM: NCsCM – 3 times (0.1-0.2 mg/ml) and 3-4 times (0.3 mg/ml, 4th-8th day), reaching 70.5% of full length of the
transection site; AMCs-CM – 1.5 times (0.1-0.2 mg/ml) and 4-7 times (0.3 mg/ml, 4th-8th day), reaching
97.4-100% of full length of the transection site. The addition of NCs CM and AMCs CM resulted in β-catenin
translocation into nucleus of cells in rat neural cell cultures, which correlated with the overgrowth of the
transection zone. NCs-CM as well as AMCs-CM in dose-dependent manner stimulate migration processes
in culture of rat neural cells, obviously, involving β-catenin signaling pathway, contributing to overgrowing
of the dissected area (reparation of a mechanical defect). NCs-CM and AMCs-CM are a source of signaling
molecules that modulate the microenvironment and activate endogenous repair mechanisms in culture (in
vitro model of nerve tissue regeneration).
Keywords:
fetal neurogenic cells; adipose-derived mononuclear cells; conditioned media; secretome; cell migration; differentiation.
References
- Ludwig PE, Thankam FG, Patil AA, Chamczuk AJ, Agrawal DK. Brain injury and neural stem cells. Neural Regen Res. 2018 Jan;13(1):7-18.
CrossRef
PubMed PubMedCentral
- Kopach O, Pivneva T. Cell-based therapies for neural replacement strategies in stroke-related neurodegeneration: neurophysiological insights into stem progenitor cell neurogenesis within a host environment. Neural Regen Res. 2018 Aug;13(8):1350-1.
CrossRef
PubMed PubMedCentral
ClinicalTrials.gov. A service of the US. National Institutes of Health. Available from: http://www: clinicaltrials.gov
- Mazini L, Rochette L, Amine M, Malka G. Regenerative capacity of adipose derived stem cells (ADSCs), comparison with mesenchymal stem cells (MSCs). Int J Mol Sci. 2019 May 22;20(10):2523.
CrossRef
PubMed PubMedCentral
- Zhou LN, Wang JC, Zilundu PLM, Wang YQ, Guo WP, Zhang SX, Luo H, Zhou JH, Deng RD, Chen DF. A comparison of the use of adipose-derived and bone marrow-derived stem cells for peripheral nerve regeneration in vitro and in vivo. Stem Cell Res Ther. 2020 Apr 9;11(1):153.
CrossRef
PubMed PubMedCentral
- Bonnamain V, Neveu I, Naveilhan P. Neural stem/ progenitor cells as a promising candidate for regenerative therapy of the central nervous system. Front Cell Neurosci. 2012 Apr 11;6:17.
CrossRef
PubMed PubMedCentral
- Kokaia Z, Martino G, Schwartz M, Lindvall O. Crosstalk between neural stem cells and immune cells: the key to better brain repair? Nat Neurosci. 2012 Jul 26;15(8):1078-87.
CrossRef
PubMed
- Haus DL, López-Velázquez L, Gold EM, Cunningham KM, Perez H, Anderson AJ, Cummings BJ. Transplantation of human neural stem cells restores cognition in an immunodeficient rodent model of traumatic brain injury. Exp Neurol. 2016 Jul;281:1-16.
CrossRef
PubMed
- Beretta S, Cunningham KM, Haus DL, Gold EM, Perez H, López-Velázquez L, Cummings BJ. Effects of human es-derived neural stem cell transplantation and kindling in a rat model of traumatic brain injury. Cell Transplant. 2017 Jul;26(7):1247-61.
CrossRef
PubMed PubMedCentral
- Walker PA, Letourneau PA, Bedi S, Shah SK, Jimenez F, Cox CS Jr. Progenitor cells as remote "bioreactors": neuroprotection via modulation of the systemic inflammatory response. World J Stem Cells. 2011 Feb 26;3(2):9-18.
CrossRef
PubMed PubMedCentral
- Wei L, Fraser JL, Lu ZY, Hu X, Yu SP. Transplantation of hypoxia preconditioned bone marrow mesenchymal stem cells enhances angiogenesis and neurogenesis after cerebral ischemia in rats. Neurobiol Dis. 2012 Jun;46(3):635-45.
CrossRef
PubMed PubMedCentral
- Walker PA, Bedi SS, Shah SK, Jimenez F, Xue H, Hamilton JA, Smith P, Thomas CP, Mays RW, Pati S, Cox CS Jr. Intravenous multipotent adult progenitor cell therapy after traumatic brain injury: Modulation of the resident microglia population. J Neuroinflammat. 2012 Sep 28;9:228.
CrossRef
PubMed PubMedCentral
- Wang S, Cheng H, Dai G, Wang X, Hua R, Liu X, et al. Umbilical cord mesenchymal stem cell transplantation significantly improves neurological function in patients with sequelae of traumatic brain injury. Brain Res. 2013 Sep 26;1532:76-84.
CrossRef
PubMed
- Webb RL, Kaiser EE, Scoville SL, Thompson TA, Fatima S, Pandya C, et al. Human neural stem cell extracellular vesicles improve tissue and functional recovery in the murine thromboembolic stroke model. Transl Stroke Res. 2018 Oct;9(5):530-9.
CrossRef
PubMed PubMedCentral
- Willis CM, Nicaise AM, Hamel R, Pappa V, PeruzzottiJametti L, Pluchino S. Harnessing the neural stem cell secretome for regenerative neuroimmunology. Front Cell Neurosci. 2020 Nov 5;14:590960.
CrossRef
PubMed PubMedCentral
- Zhong D, Cao Y, Li CJ, Li M, Rong ZJ, Jiang L, et al. Neural stem cell-derived exosomes facilitate spinal cord functional recovery after injury by promoting angiogenesis. Exp Biol Med (Maywood). 2020 Jan;245(1):54-65.
CrossRef
PubMed PubMedCentral
- Xu C, Diao YF, Wang J, Liang J, Xu HH, Zhao ML, et al. Intravenously infusing the secretome of adipose-derived mesenchymal stem cells ameliorates neuroinflammation and neurological functioning after traumatic brain injury. Stem Cells Dev. 2020 Feb 15;29(4):222-34.
CrossRef
PubMed
- Kocan B, Maziarz A, Tabarkiewicz J, Ochiya T, BanaśZąbczyk A. Trophic Activity and phenotype of adipose tissue-derived mesenchymal stem cells as a background of their regenerative potential. Stem Cells Int. 2017;2017:1653254.
CrossRef
PubMed PubMedCentral
- Harrell CR, Fellabaum C, Jovicic N, Djonov V, Arsenijevic N, Volarevic V. Molecular mechanisms responsible for therapeutic potential of mesenchymal stem cell-derived secretome. Cells. 2019 May 16;8(5):467.
CrossRef
PubMed PubMedCentral
- Xing X, Han S, Cheng G, Ni Y, Li Z, Li Z. Proteomic analysis of exosomes from adipose-derived mesenchymal stem cells: A novel therapeutic strategy for tissue injury. Biomed Res Int. 2020 Mar 3;2020:6094562.
CrossRef
PubMed PubMedCentral
- Lee EM, Kim JY, Cho BR, Chung WK, Yoon BW, Kim SU, et al. Down-regulation of MHC class I expression in human neuronal stem cells using viral stealth mechanism. Biochem Biophys Res Commun. 2005 Jan 28;326(4):825-35.
CrossRef
PubMed
- Jacobs SA, Roobrouck VD, Verfaillie CM, Van Gool SW. Immunological characteristics of human mesenchymal stem cells and multipotent adult progenitor cells. Immunol Cell Biol. 2013 Jan;91(1):32-9.
CrossRef
PubMed PubMedCentral
- Ubiali F, Nava S, Nessi V, Frigerio S, Parati E, Bernasconi P, Mantegazza R, Baggi F. Allorecognition of human neural stem cells by peripheral blood lymphocytes despite low expression of MHC molecules: role of TGFbeta in modulating proliferation. Int Immunol. 2007 Sep;19(9):1063-74.
CrossRef
PubMed
- Vagaska B, New SE, Alvarez-Gonzalez C, D'Acquisto F, Gomez SG, Bulstrode NW, Madrigal A, Ferretti P. MHC-class-II are expressed in a subpopulation of human neural stem cells in vitro in an IFNγ-independent fashion and during development. Sci Rep. 2016 Apr 15;6:24251.
CrossRef
PubMed PubMedCentral
- Barrachina L, Cequier A, Romero A, Vitoria A, Zaragoza P, Vázquez FJ, Rodellar C. Allo-antibody production after intraarticular administration of mesenchymal stem cells (MSCs) in an equine osteoarthritis model: effect of repeated administration, MSC inflammatory stimulation, and equine leukocyte antigen (ELA) compatibility. Stem Cell Res Ther. 2020 Feb 7;11(1):52.
CrossRef
PubMed PubMedCentral
- Rowland AL, Miller D, Berglund A, Schnabel LV, Levine GJ, Antczak DF, Watts AE. Cross-matching of allogeneic mesenchymal stromal cells eliminates recipient immune targeting. Stem Cells Transl Med. 2021 May;10(5):694-710.
CrossRef
PubMed PubMedCentral
- Berglund AK, Long JM, Robertson JB, Schnabel LV. TGF-β2 reduces the cell-mediated immunogenicity of equine MHC-mismatched bone marrow-derived mesenchymal stem cells without altering immunomodulatory properties. Front Cell Dev Biol. 2021 Feb 4;9:628382.
CrossRef
PubMed PubMedCentral
- Rybachuk O, Kopach O, Pivneva T, Kyryk V. Isolation of Neural Stem Cells from the embryonic mouse hippocampus for in vitro growth or engraftment into a host tissue. Bio Protoc. 2019; 9(4): e3165.
CrossRef
PubMed PubMedCentral
- Lööv C, Hillered L, Ebendal T, Erlandsson A. Engulfing astrocytes protect neurons from contact-induced apoptosis following injury. PLoS One. 2012;7(3):e33090.
CrossRef
PubMed PubMedCentral
- Clevers H. Wnt/beta-catenin signaling in development and disease. Cell. 2006 Nov 3;127(3):469-80.
CrossRef
PubMed
- Klaus A, Birchmeier W. Wnt signalling and its impact on development and cancer. Nat Rev Cancer. 2008 May;8(5):387-98.
CrossRef
PubMed
- Nusse R. Wnt signaling and stem cell control. Cell Res. 2008 May;18(5):523-7.
CrossRef
PubMed
- Thrasivoulou C, Millar M, Ahmed A. Activation of intracellular calcium by multiple Wnt ligands and translocation of β-catenin into the nucleus: a convergent model of Wnt/Ca2+ and Wnt/β-catenin pathways. J Biol Chem. 2013 Dec 13;288(50):35651-9.
CrossRef
PubMed PubMedCentral
- Lien WH, Fuchs E. Wnt some lose some: transcriptional governance of stem cells by Wnt/β-catenin signaling. Genes Dev. 2014 Jul 15;28(14):1517-32.
CrossRef
PubMed PubMedCentral
- Kaldis P, Pagano M. Wnt signaling in mitosis. Dev Cell. 2009 Dec;17(6):749-50.
CrossRef
PubMed
- Zou Y. Wnt signaling in axon guidance. Trends Neurosci. 2004 Sep;27(9):528-32.
CrossRef
PubMed
- Salinas PC. Synaptogenesis: Wnt and TGF-beta take centre stage. Curr Biol. 2003 Jan 21;13(2):R60-2.
CrossRef
- Hall AC, Lucas FR, Salinas PC. Axonal remodeling and synaptic differentiation in the cerebellum is regulated by WNT-7a signaling. Cell. 2000 Mar 3;100(5):525-35.
CrossRef
- Chin J, Angers A, Cleary LJ, Eskin A, Byrne JH. Transforming growth factor beta1 alters synapsin distribution and modulates synaptic depression in Aplysia. J Neurosci. 2002 May 1;22(9):RC220.
CrossRef
PubMedCentral
|